Groundwater
Tracing in the Woodville Karst Plain - Part I:
An Overview of Groundwater Tracing
Source:
DIR Quest (Journal of the Global Underwater Explorers), Vol. 4, No. 4 Fall
2003, p. 31-37, High Springs, Florida.
BACKGROUND
The purpose of this presentation is to provide an overview of recent groundwater tracing efforts in North Central Florida that have been undertaken as part of a broader effort to protect the water quality in Wakulla Spring and a discussion of some implications to development strategies and land use practices and that have arisen from these efforts. The specific tracer tests that will be discussed have been made possible by GUE support and its WKPP project divers. Funding support was provided by the Florida Geological Survey (FGS) and carried out by GUE VP/Science director Dr. Todd Kincaid in cooperation with H2H Associates and the Florida State University Oceanography Department in a cooperative effort led by the Florida State University Geophysical Fluid Dynamics Institute and the Hydrogeology Consortium.
|
Floridan
Aquifer
The Floridan aquifer encompasses all of the water bearing limestone
rocks in Florida that contain the underwater cave systems familiar
to cave divers around the world including the massive caves in the
Woodville Karst Plain. Hydrogeologically speaking, the Floridan
aquifer is in a special class of aquifers known as karst, which,
loosely defined, describes aquifers made of soluble rock wherein
groundwater flow dissolves preferential flow paths (caves) that then
control the flow rate and direction. Unlike most of the rest of the
karst aquifers in the world (e.g. the limestone rocks containing the
big dry caves in the Appalachian Mountains, Mammoth Cave, Carlsbad
Caverns, etc.), the Floridan aquifer is further unique because the
limestone rocks comprising the aquifer are themselves highly
permeable. This means that groundwater, in usable quantities, can be
found almost anywhere in Florida, not just in the caves, as is most
often the case in other karst settings.
The
unique hydrologic conditions that characterize the Floridan aquifer
(karstic features within a highly permeable aquifer matrix) have
been both blessing and curse to the preservation of Florida's
natural resources. Blessing, because development has not, until the
recent boon in the water bottling industry, needed to focus on
Florida's springs or rivers as a major source of fresh water. There
are very few reservoirs in Florida,
|
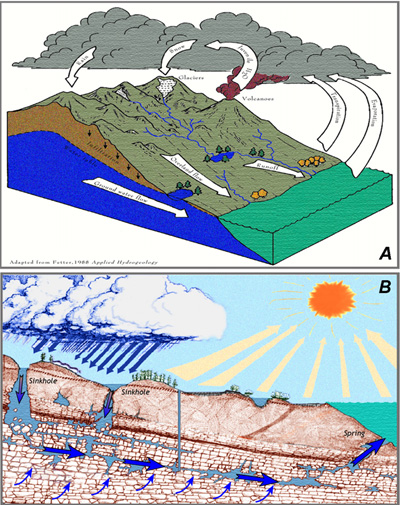 Figure
1.
Generalized
conceptualization of the hydrogeologic cycle showing water in
constant circulation between the atmosphere, surface water, and
groundwater. (A) Most common conceptualization of the groundwater
reservoir indicating that indicating that aquifers are an
essentially uniform mass of porous material wherein groundwater
moves very slowly down-gradient toward lakes, rivers, and streams.
(B) Refined conceptualization of karst aquifers wherein dissolved
conduits and caves carry the majority of the groundwater very
rapidly to spring discharges. |
and no
springhead pipelines to speak of, thereby leaving the vast majority
of Florida's natural hydrologic resources open and untouched by
industrial development. In fact, very few people and none of the
current legislation consider Florida's springs and rivers as
significant sources of potable water for either industrial or public
supply. Instead, the permeable nature of the Floridan aquifer has
long been an adequate provider of Florida's fresh water needs
meaning that water supply is largely from wells drilled in locations
that are chosen more for the logistics of delivery than for the
productivity of the aquifer. |
Poor
Scientific Assumptions
Unfortunately, the same conditions that have enabled development to
proceed nearly oblivious to abundant water resources provided by
Florida's springs and rivers has also been, and continues to be, a
curse to resource protection efforts in more subtle ways. The curse
arose and flourishes on a fundamental misunderstanding of the
significance of caves and other karst features in Florida to
groundwater flow patterns and velocities. Basically, because highly
productive wells can be drilled at almost any location in Florida,
hydrogeologists and water resource managers, most having never been
in an underwater cave in Florida, were quick to assume that such
features were anomalous and not pertinent to groundwater flow
patterns. This was an understandable assumption given the comparison
of Florida to other, recognized, karst settings where water wells
are typically either dry or deliver very low volumes of water when
they do not intersect caves or dissolved fractures. In a practical
sense, the simplifying assumption applied to the Floridan aquifer is
that groundwater flow is largely diffuse and through a porous media
with a few discrete discharge locations (springs); rather than the
more complicated, though valid condition, where flow through the
diffuse media converges on progressively larger conduits that
deliver the water to the springs (Figure 1). This assumption
persisted largely unchallenged until recently when the decline in
spring water quality across Florida due to increased nitrate levels
became all but undeniable and water resource managers were forced to
consider that the traditional assumptions about the Floridan aquifer
might be wrong; that caves do exist, are more common than earlier
assumed, and are probably important pathways for contamination. |
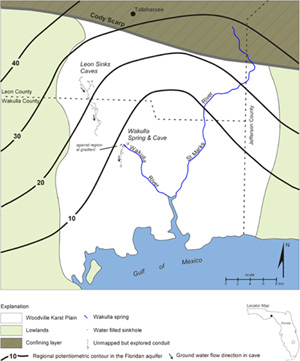
Figure 2: Map of the
Woodville Karst Plain, north central Florida showing the location of
the Leon Sinks cave system in the north and Wakulla cave in the
south relative to the position of the Cody Scarp, which demarks the
transition from confined aquifer conditions in the north to
unconfined conditions in the south. Note that the potentiometric
surface lines, which are drawn assuming that there are no caves in
the region, indicate a general flow to the south rather than
concentrated flow in the caves. |
The
Woodville Karst Plain
The Woodville Karst Plain (WKP) of north Florida, though it
contains two of the largest springs in Florida and two of the
largest and longest mapped underwater cave systems in the world, is
one of the many karst basins in Florida where the simplifying
assumption about groundwater flow has led to a poor understanding of
the realities of groundwater flow patterns, rates, and their impact
on spring water quality.
The WKP is a
broad lowland that extends from just south of Tallahassee to the
Gulf of Mexico, where the Floridan aquifer is either unconfined or
poorly confined (Figure 2). The region is underlain by numerous very
large underwater cave systems that convey water from upland recharge
areas to spings such as Spring Creek and Wakulla springs, the
largest and third largest springs in Florida respectively, and
Indian, Sally Ward, and Mc Brides springs. Underwater cave surveys
conducted by the WKPP indicate that the Leon Sinks cave system,
alone, contains more than 18 km of saturated conduits that convey
groundwater generally north-south through a series of 26 sinkholes
or "karst windows" toward the Gulf of Mexico. The
up-gradient most sinkholes are located in the Leon Sinks Geological
Park in Leon County. The down-gradient most sinkhole is Turner Sink
located in Wakulla County northwest of the town of Bethel between
State Road 267 and State Road 61. A large conduit approximately 15 m
in diameter has been explored and mapped for approximately 1.2 km
further down-gradient and appears to continue without any
diminishment of size or flow (Jablonski, personal communication).
Discharge from the system is thought to occur at either at Wakulla
spring or, more probably, the Spring Creek springs located on the
Gulf Coast. Wakulla Cave contains an additional 10-12 km of
underwater conduits, the largest of which trend for more than 6 km
south of Wakulla Spring (Kincaid, 2000). Conduit diameters
throughout these caves range from less than 2 meters to more than 30
meters and average approximately 10-15 meters. The average depth of
the conduits is approximately 85 meters below the water table
surface. Flow rates through the conduits have not been directly
measured, however the velocities are sufficiently large to impede a
divers ability to swim against the flow direction even in the
largest passages.
Considering
these characteristics, it becomes evident that the caves are the
most significant hydrogeologic features in the WKP, however there
remains very little substantive scientific information about the
caves or how they affect groundwater flow patterns in the region, or
how they contribute to the increasing water quality problems at the
springs. To address this deficiency, the Florida Geological Survey
initiated a comprehensive research program aimed at characterizing
groundwater flow paths through the WKP in the summer of 2002 that
includes the tracer testing efforts described in this paper. |
GROUNDWATER TRACING - AN OVERVIEW
Groundwater tracers include any substance that can become
dissolved or suspended in water, or attached to the water molecule,
and recovered or measured from a water sample that can be used to
trace the source of groundwater in terms of its specific or relative
location and time of recharge. Groundwater tracers can include both
artificially introduced and naturally occurring substances.
Groundwater tracing as a science has been in practice for more than
a century but has been slow to become accepted in the United States
largely because karst aquifers have traditionally received little
attention from the traditional hydrogeological communities.
Artificial
Tracers
Groundwater tracing with artificial tracers involves adding a label
to the groundwater that can be identified if that same water is
sampled at a different location. Tracing involves making almost no
assumptions about an aquifer's hydraulic properties; the principal
assumption made is that the tracer can be identified when it is
recovered. When used properly, artificial tracers, therefore,
provide the most reliable data on groundwater flow patterns and
velocities that can be obtained. The most commonly used artificial
tracers are fluorescent dyes such as: Rhodamine WT (red), Eosin
(green), Uranine (green), and optical brighteners (various colors),
however many other substances have been used including: chlorine
(salt), low level radioactive compounds, bacterial phage,
microspheres (tiny plastic balls), ping-pong balls, etc. Contaminant
spills can also be used as artificial tracers if the source and
timing of the spill can be confidently documented. The purpose of
using artificial tracers is to answer three basic questions. 1.
Where does the water go (say from a sinkhole) or where does the
water come from (say from a spring)? 2. How long does it take to get
there? 3. What happens to the water along the way? |
Fluorescent Dyes
Fluorescent dyes are some of the oldest groundwater tracers known
and have been used successfully for more than 100 years. A
quantitative tracer test was done using the fluorescent tracer dye
uranine in 1877 between the Rhine River and the Aäch Spring near
the Swiss border, which showed that there was a reversal of flow of
the Danube River across the European Continental Divide (Käss,
1998, p. 125). Since then more than 20,000 professionally conducted
tracer tests using fluorescent dyes have been conducted worldwide,
most of them in karst terrains. Fluorescent dyes are now the most
commonly used groundwater tracers because they fulfill the following
criteria for optimal artificial tracers:
-
they
are readily soluble in water;
-
adequately conservative (they don't react with water or soil/rock);
-
unambiguously and inexpensively detectable at very small concentrations;
-
readily
available and relatively inexpensive; and, most importantly
-
intrinsically
low in toxicity thereby posing no significant health or
environmental hazard (except for pink or green fingers).
When
a fluorescent dye is injected or released into a water body it mixes
with the water, travels downstream with the flow and spreads due to
molecular diffusion. At and near the point of injection, even very
small quantities of a fluorescent dye will appear to stain the water
yielding a vivid coloration (Figure 3). When small quantities are
used however, the concentration of the |
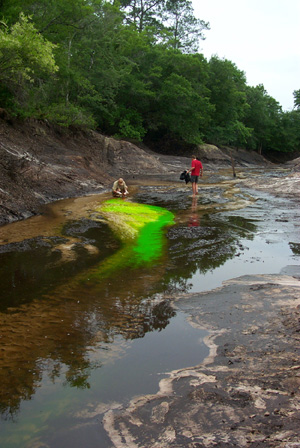
Figure 3: Uranine dye
moving downstream in Fisher Creek during a 2003 injection to trace
the destination of the water disappearing at Fisher Creek. |
dye will quickly fall below the visible
detection limit downstream of the injection point. Sampling is
therefore conducted using a fluorometer, which measures the
fluorescence of a water sample. Detection of the dye is therefore
predicated on the ability to measure an increase in fluorescence in
the water. Waters with low and consistent background fluorescence,
typical of most groundwater, are therefore best suited for dye
tracing. |
Qualitative
Tracer Tests
There are two basic types of dye tracer tests: qualitative and
quantitative. Qualitative tests are the simplest, most common, but
least informative. They are designed to answer the basic question of
connection. One or more dyes are injected at an upstream location(s).
Samples are collected at downstream locations. If the dye is
observed, a connection is verified and an approximate travel time
can be determined. Typically, qualitative tests are conducted using
some type of dye trap, such as activated charcoal or cotton, at the
sampling locations. The traps, or bugs, are often left for long
periods of time, after which any dye is eluded from the absorptive
material into a water sample and tested with a fluorometer to
determine if any dye is present in the sample. Simplicity is the
most significant advantage of a qualitative test wherein many
locations can be sampled with minimum resources. Problems with
quantitative testing revolve around control because, over broader
time periods, it is often impossible to ensure that your dye is the
only fluorescent substance introduced to the flow system or that
small spikes in fluorescence are not simply due to fluctuations in
the background levels. |
Quantitative
Tracer Tests
Quantitative tests, on the other hand, are more complex to design,
more labor intensive to conduct, but deliver far more useful and
reliable information. A quantitative test depends not only on the
detection of a fluorescent substance at the sampling locations but
rather on the observation of the trend in fluorescence (from
background levels to peak concentration and back to background
levels) as the dye passes the observation point. The observations
are recorded on a plot of the fluorescence or dye concentration
levels over time, which is often called a breakthrough curve (Figure
4). The breakthrough curve offers many benefits over a single test
including:
|
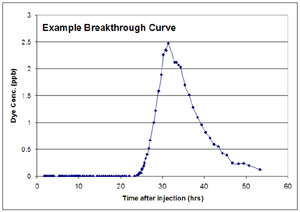
Figure 4: Example
breakthrough curve for an injected dye tracer. The width of the
curve provides information about dispersion and sinuosity of the
conduit flow path. Groundwater velocities are calculated from the
time at which the peak concentration passes the sampling station. |
-
a more accurate
groundwater velocity can be calculated using the time-to-leading
edge and time-to-peak concentrations; and
-
evaluating the
shape and integrating the trend of the concentration vs. time
plot provides for the calculation of other hydraulic parameters
including: longitudinal dispersion, Reynolds and Peclet Numbers,
and discharge.
Quantitative
tracers tests can be conducted wherein recovery curves have a peak
concentration of less than one part per billion, which is far below
the visible detection limit. However, in order to achieve successful
tests, sampling strategies must be designed such that numerous
samples are collected from within the dye cloud as it passes a
sampling point. Such sampling strategies are typically laborious and
their design often requires a fair amount of professional judgment
and luck.
Natural
Tracers
Natural tracing involves the use of naturally occurring components
of a water sample to determine information about the source and age
of the sample. The most commonly used natural tracers are isotopes
and chemical compounds that originate in the atmosphere and become
incorporated in the rainfall the recharges an aquifer. Of those
types of substances, the isotopes of oxygen (16O/18O)
and hydrogen (2H/3H), and chlorofluorocarbons
(CFC) are the most commonly used tracers today. Isotopes of other
elements such as Radon, sulfur, chlorine, lead, strontium, helium
and carbon are other notable tracers that are fairly common in
hydrogeology.
In
the case of age-dating, the basic idea behind the use of natural
tracers is that of a two-component mixing model, wherein the
concentrations of a given substance can be fairly well constrained
in two separate source waters and some value in between the two end
members represents a mixture of only those two waters. Knowing
something about decay rates or linear changes in the source
concentrations provides for an estimation of age, or time since
recharge.
For
example, tritium (3H), which has been one of the most
commonly used radiogenic isotopes, was released to the atmosphere in
large quantities during the atmospheric nuclear testing program,
which largely began in the 1950's and peaked in the early 1960's.
During the peak period, atmospheric nuclear explosions typically
released several hundred tritium units (TU) to the atmosphere that
was eventually incorporated into precipitation. The dramatic
increase in atmospheric TU during this period is often referred to
as the "bomb spike" and is the reason that TU can be used
as an effective groundwater tracer.
After
the end of the atmospheric testing program the source of tritium to
the atmosphere was eliminated and, because tritium has a relatively
short half-life (12.3 years), there has been a predictable decline
in TU concentrations in groundwater. After accounting for
radioactive decay, water with TU values similar to atmospheric
levels during the bomb spike can be attributed to recharge during
that period. As time goes by, the usefulness of TU as a groundwater
tracer will decline because radioactive decay will render the TU
values of bomb spike water similar to the current atmospheric
levels. Raw TU values continue to be useful as a tracer because the
tritium activity has not yet decayed away to completely undetectable
amounts, although in many parts of the country, including Florida,
the TU values are approaching undetectable levels (Clark and Fritz,
1997).
The
main problem with the use of natural tracers to estimate the age of
a water sample is the often erroneous, 2-component mixing model
assumption, i.e. one source of recharge to one groundwater body. In
the case of tritium, any TU number that is not either clearly
related to the bomb spike or pre-bomb testing could be any mixture
of several waters of different aquifer residence times. The utility
of the TU as a quantitative tracer is thus severely limited in
regions where groundwater discharge is known to be a composite of
waters from multiple sources, which is likely the case in the karst
regions of Florida. The same problem plagues all of the natural
tracers as tools for calculating the age of any water sample
especially in the Floridan aquifer, where spring discharges are
known to be a mixture of, at very least, older matrix water and more
recent recharge that enters the aquifer through discrete sources
such as sinkholes. This problem will be explored in more detail in
the discussion of tracing in the Woodville Karst Plain. |
WHY
IS TRACING IMPORTANT?
Florida's spring water quality has been declining markedly for more
than 10 years, with the most notable problem being increasing
nitrates. Within the last 5 years, the increasing nutrient levels
has led to obvious and alarming changes in the water clarity and
ecology of many spring basins. At Catfish Hotel, the most popular
entrance to the Manatee Springs Cave System, dark brown algae covers
the limestone walls in the sinkhole basin and near-entrance cave
walls, which used to be clean rock surfaces. The same conditions are
emerging at Devil's Eye and Ear, Blue Springs Orange City, Indian,
Wakulla, and many more. If the trend continues, it is doubtful that
any of us will ever again behold the air-clear conditions that used
to typify most of Florida's springs and spring caves, as particulate
levels continue to climb due to the increase in algae and bacteria
in the water.
Though
these statements are fact, they are all too often dismissed as
environmental alarmism or worse, inevitabilities. There is however,
another perspective that serves as motivation for change. Consider
that Florida |
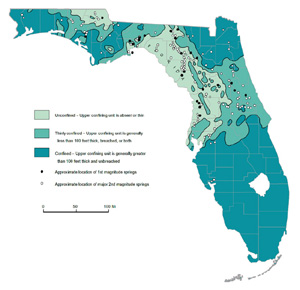
Figure
5: Map showing the distribution of springs in Florida relative to
aquifer confinement. Note that most of the springs cluster in the
regions where the aquifer is either unconfined or thinly confined. |
boasts the highest
concentration of springs on the planet including 33 documented
first-magnitude (very big) springs and more than 600 2nd and 3rd
magnitude springs. Most of these springs are concentrated in a
fairly small part of the state where the Floridan aquifer is
unconfined (Figure 5); an area that extends like a crescent from
Clearwater in the southwest up through the center of the peninsula
and west across the panhandle to the Woodville Karst Plain. Until
recently, most of these springs typically discharged crystal clear,
pure fresh water to the land surface. People drank the water without
treatment. Divers enjoyed visibility conditions limited only by the
power of their lights. But, perhaps most significantly, people have
long been coming from literally around the world to experience the
springs because they know and have known what many Floridians have
missed, that these springs make Florida unique, that such an
abundance of clear fresh water cannot be found anywhere else on
Earth. So, not only are we loosing our clear freshwater dive spots,
we're systematically loosing the one place on Earth where such
conditions could be found in abundance. References
Clark,
I.D., and Fritz, P., 1997, Environmental Isotopes in Hydrogeology,
Lewis, New York, 328 p.
Jablonski,
J.M., 2002, personal communication; Global Underwater Explorers, 15
south Main St., High Springs, Florida; phone (386) 454-0811.
Käss,
W., 1998, Tracing Technique in Geohydrology, Balkema, Rotterdam, 581
p.
Further
Reading
Cao,
H., J. Cowart, and J. Osmond, n.d., Water Sources of Wakulla
Springs, Wakulla County, Florida: Physical and Uranium Isotopic
Evidences, Tallahassee, FL, 12 pages.
Field,
M., R. Wilhelm, J. Quinlan and T. Aley, 1995, An Assessment of the
Potential Adverse properties of Fluorescent Dyes used for
Groundwater Tracing, Environmental Monitoring and Assessment, vol.47
pp. 1-21.
Field,
M. and S. Nash, 1997, Risk Assessment Methodology for Karst
Aquifers: (1) Estimating Karst Conduit-flow Parameters,
Environmental Monitoring and Assessment, vol.35 pp. 75-96.
Hisert,
R.A., 1994, A multiple tracer approach to determine the ground and
surface water relationships in the western Santa Fe River, Columbia
County, Florida, Ph.D. Dissertation, University of Florida,
Gainesville, Florida.
Katz,
B., T. Coplen, T. Bullen, and J. Davis, 1997, Use of Chemical and
Isotopic Traces to Characterize the Interactions between Ground
Water and Surface Water in Mantled Karst, Groundwater, vol. 26, no.
6, pp.1014-1028
Katz,
B., J. Catches, T Bullen, and R. Michel, 1998, Changes in the
isotopic and chemical compostion of ground water resulting from a
recharge pulse from a sinking stream, Journal of Hydrology, Vol.
211, pp. 178-207.
Kincaid,
T., 1998, River Water Intrusion to the Unconfined Floridan Aquifer,
Environmental & Engineering Geoscience, vol. 4, no.3, pp.
361-374.
Quinlan,
J and A. Stanley, 1992, The Reponse of Landfill Monitoring Wells in
Limestone (Karst) Aquifers to point sources and non point sources
contamination, Conference on Hydrogeology, Ecology, Monitoring, and
Management of Grounwater in Karst Terranes, Nashville, TN,
Proceedings, National Groundwater Association, Dublin, OH,
pp.529-540.
Skiles,
W., Hayes, A.H., and Butt, P.L., 1991, Ichetucknee Hydrogeology
Study, Florida Department of Natural Resources, Florida Park
Service, District III, Gainesville, Florida.
Wilson,
W.L. and Skiles, W.C., 1988, Aquifer characterization by
quantitative dye tracing at Ginnie Spring, northern Florida, The
Proceedings of the Second Conference on Environmental Problems in
Karst Terranes and Their Solutions, The Association of Groundwater
Scientists and Engineers, Nashville, TN.
Wilson,
W.L., 1991, Dye Tracing Benefits and Practice in Florida, NACD
Journal, vol. 23, no. 2.
|
|